Offshore wind, solar, and hydrogen systems are at the forefront of this transition, but the scale and complexity of these projects present significant challenges. From permitting delays to fragmented compliance workflows, inefficiencies threaten the sector’s ability to meet its goals.
While permitting bottlenecks remain critical — potentially reducing global offshore wind installations by 30% this decade, according to the DNV Energy Transition Outlook 2023 — they are only part of the problem. Inflation, supply chain disruptions, and evolving regulatory standards add further strain. Fragmented systems and outdated workflows exacerbate delays and inflate costs, especially in offshore wind projects where timelines often exceed 10 years.
The technical demands of renewable energy projects also highlight a major bottleneck: compliance workflows. Engineers must navigate disconnected tools — switching between CAD models, finite element analysis (FEA) platforms, and manual reporting. This fragmented approach hampers efficiency and increases errors. For example, hydromechanical challenges in floating wind systems, including coupled wind-wave-current interactions, require precise workflows that traditional methods cannot deliver.
Without addressing these inefficiencies, renewable energy projects risk falling behind, jeopardising decarbonisation targets and stalling industry growth.
Why Current Workflows Fail
Traditional compliance workflows in renewable energy projects rely heavily on manual processes and outdated tools, which are increasingly inadequate for the complexity of modern designs. Engineers manually interpret finite element analysis (FEA) outputs to validate structural elements against standards such as DNV, ISO, and AISC, introducing inefficiencies and risks, including human error, oversight, and inconsistencies.
Hydromechanical Complexity Floating wind turbines and hydrogen production systems present unique challenges. The distribution of platform mass, resonance effects, and interactions between wind, wave, and current forces demand precise modelling and validation.
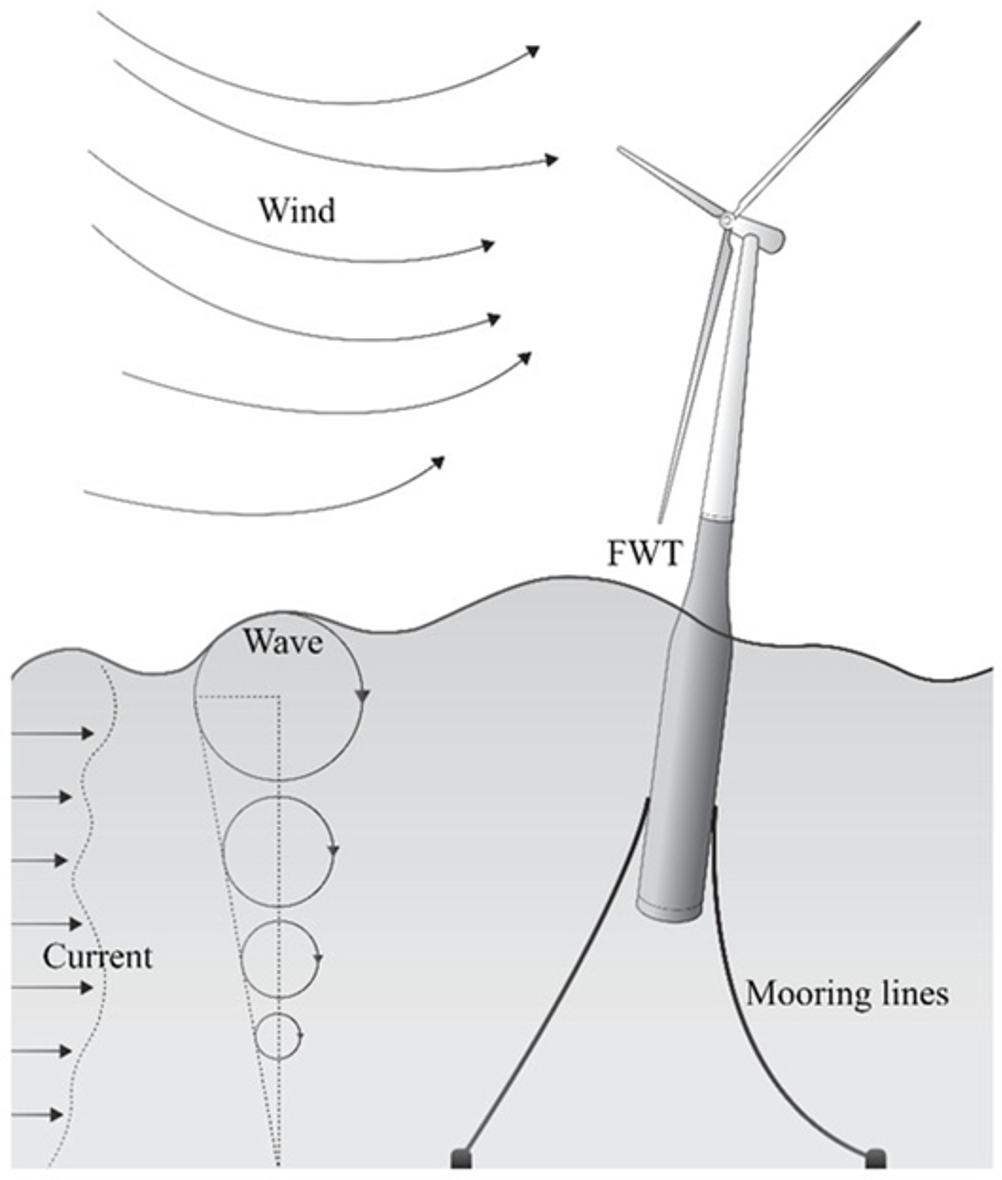
Manual workflows often fail to account for critical factors, such as liquid motion in storage tanks or extreme weather survivability, leading to designs that are inefficient or non-compliant. For example, coupled wind-wave-current interactions require detailed, fully coupled simulations—something manual methods struggle to handle effectively.
Fatigue Analysis Time-domain simulations, essential for assessing coupled wind and wave dynamics, are computationally intensive and prone to error when performed manually. Fatigue hotspots in welded tubular joints, exacerbated by dynamic offshore environments, require detailed analysis across hundreds of load cases. Studies confirm that traditional workflows lack the precision and efficiency to address these demands comprehensively.
Corrosion Risks Corrosion in splash and tidal zones is a significant challenge, particularly for offshore wind structures operating in corrosive environments. Without probabilistic models to predict corrosion rates and their impact on structural fatigue, traditional workflows risk underestimating long-term vulnerabilities, compromising safety and durability. Improved modelling techniques are urgently needed to mitigate these risks effectively.
Impact of Workflow Inefficiencies
· Delays and Rework: Fragmented workflows, exacerbated by permitting delays and interconnection challenges, significantly prolong project timelines and increase costs.
· Overengineering: To compensate for uncertainties, engineers overdesign structures, resulting in material waste and inflated budgets.
· Sustainability Gaps: Inefficient processes lead to excessive resource use, contradicting the renewable energy sector’s environmental objectives.
The Case for Automation
Manual compliance workflows in renewable energy projects are no longer sufficient to handle the scale, speed, and complexity of modern offshore wind systems. Automation provides a scalable, precise solution by integrating advanced tools that streamline validation, improve accuracy, and reduce inefficiencies.
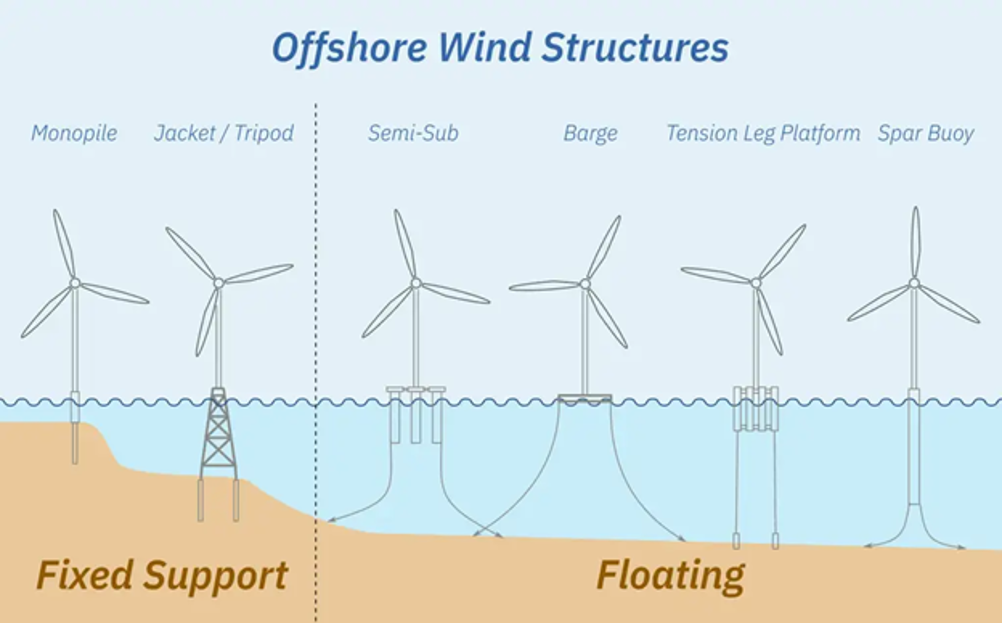
Automated Detection and Validation
Manual identification of critical structural components — welds, joints, and plates — within finite element analysis models is not only slow but also highly susceptible to errors. Given the complexity of wind turbine models, subtle yet critical results can often be overlooked, leading to incomplete assessments and potential compliance risks.
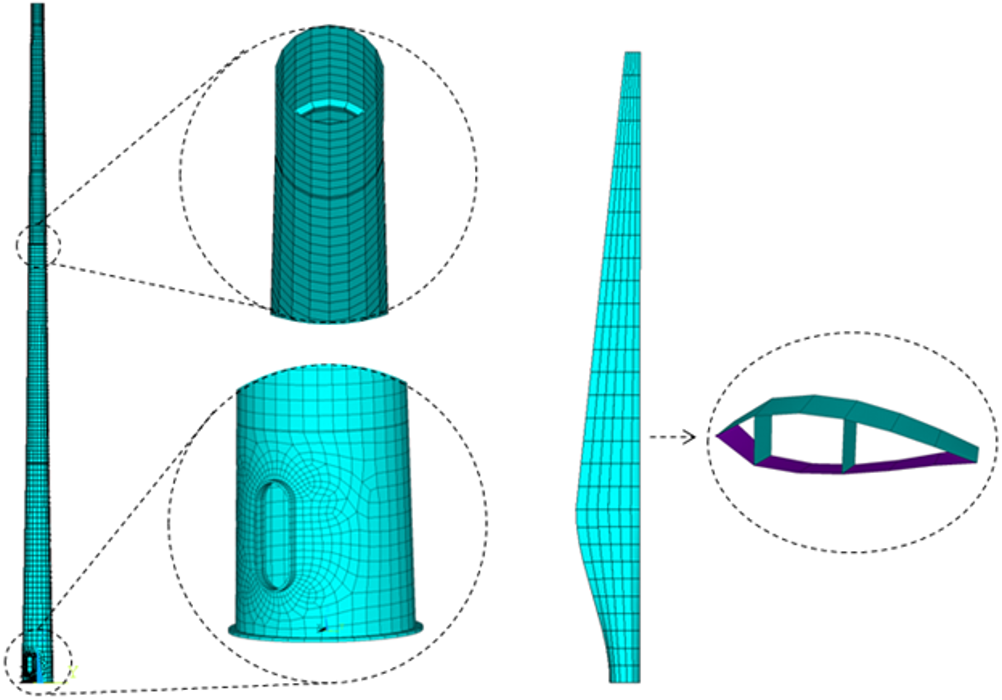
SDC Verifier, a type of structural design and analysis software, automates this process, ensuring accurate detection and seamless integration of complex standards like DNV-RP-C203 for fatigue or ISO 19902 for offshore structures. Automation eliminates human oversight while improving efficiency and compliance precision.
Digital Twins
Digital twin technology is transforming compliance workflows by creating dynamic, real-time replicas of offshore wind structures. By integrating live monitoring data with advanced FEA models, digital twins enable predictive validation of structural fatigue, hydromechanical responses, and corrosion risks. For instance, they allow engineers to simulate wind-wave-current interactions in floating wind turbines and predict failure points, improving risk management and reducing costly rework.
AI-Driven Optimization
Artificial intelligence and machine learning play a pivotal role in automating compliance processes. AI-driven tools analyze massive datasets to identify patterns, assess fatigue hotspots, and optimize designs for material efficiency. For example, spectral-based fatigue methods powered by AI models can drastically reduce computational effort compared to traditional time-domain simulations, delivering faster results without compromising accuracy.
Multi-Standard Compliance
Renewable energy projects must comply with overlapping standards (DNV, ISO, API). Manual workflows struggle to meet this demand efficiently. Automated solutions validate designs against multiple standards simultaneously, streamlining the process. For example, jacket-type support structures in offshore wind projects require compliance checks for local buckling, fatigue damage, and ultimate limit state (ULS) — all of which can be automated, ensuring consistent results.
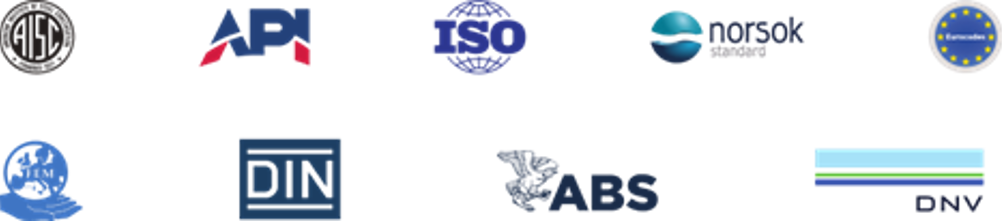
Why It Matters
Automation does not replace engineering judgment; it enhances it. By integrating tools like SDC Verifier, engineers can focus on innovation rather than repetitive tasks. The result is faster compliance validation, improved structural accuracy, and significant cost savings.
Addressing Common Concerns
Adopting automation often faces resistance due to misconceptions about safety, cost, and accessibility. Addressing these concerns is essential for broader acceptance among engineers and stakeholders in the renewable energy sector.
Myth 1: Manual Is Safer
Some believe manual compliance checks ensure better oversight and fewer errors. However, manual processes are inherently susceptible to oversight, inconsistency, and errors, particularly in repetitive tasks such as identifying welds or verifying buckling thresholds. Automation eliminates these risks through precision and consistency, enabling engineers to focus on higher-value decision-making. With the increasing complexity of offshore systems — such as floating turbines exposed to extreme weather and hydrodynamic forces — manual oversight often fails to detect subtle design vulnerabilities.
Myth 2: Automation Is Expensive
The upfront costs of automation tools are often overstated compared to their long-term value. Studies have shown significant cost reductions when automating compliance processes. For instance, proactive transmission planning in offshore wind projects reduces costs by up to 50% compared to incremental approaches. Automation similarly streamlines workflows, reduces rework, and optimizes material usage, leading to measurable savings across project lifecycles.
Myth 3: Steep Learning Curve
Modern automation tools, like SDC Verifier, are designed for ease of integration with existing finite element analysis (FEA) platforms. User-friendly interfaces and guided workflows minimize training time and disruption. Teams can adopt these tools incrementally, avoiding productivity losses. Industry examples show that engineers quickly adapt to automated tools when workflows are simplified and the benefits — such as faster compliance checks and reduced manual tasks — are clear.
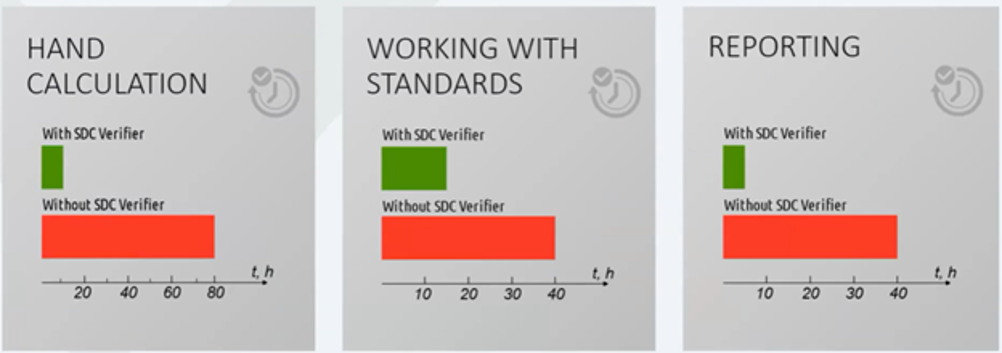
Engineers Need to Work Smarter, Not Harder
The rapid expansion of renewable energy requires modern compliance workflows that can manage increasing complexity and scale. Traditional manual methods are no longer sufficient — they lead to delays, overengineering, and rising costs, threatening the sector's ability to meet ambitious decarbonization goals.
According to the DOE Offshore Wind Market Report 2023, proactive investments in technology, including automated workflows, significantly accelerate project timelines and reduce material waste.
Modern workflows also support sustainability objectives. For instance, integrated systems help optimize resource use, cutting material waste and supporting renewable energy's broader environmental goals. This alignment is vital as the industry strives to balance rapid growth with ecological responsibility.
The future of renewable energy lies in smarter workflows. Now is the time to embrace these changes and build the next generation of sustainable energy systems.
Radio wave weapon knocks out drone swarms
Probably. A radio-controlled drone cannot be completely shielded to RF, else you´d lose the ability to control it. The fibre optical cable removes...