There’s a small part of Oxfordshire where you can go from archetypal rural idyll to the heat of the heart of a star within 10 minutes. Thatched cottages, tranquil streams and signs to the World Poohsticks Championship give way to security barriers, utilitarian buildings and a towering fortress-like structure: the Culham Innovation Centre, one of the world’s leading centres for nuclear fusion research.
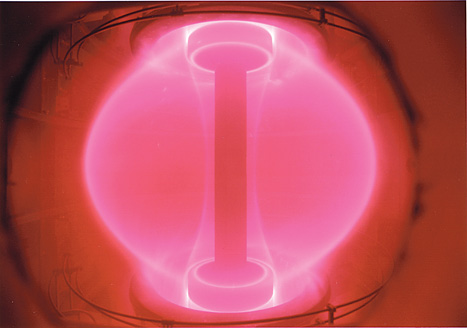
The fortress contains JET, the Joint European Torus, one of the few places on the planet capable of carrying out nuclear fusion. JET is the model for ITER, the much larger toroidal reactor whose construction is in its early stages in southern France and that, in 10 years, may point the way to one of science and engineering’s most promising but elusive goals: generating clean energy from nuclear fusion.
But energy isn’t the only product of the process that takes place inside fusion reactors; the joining together of two forms of hydrogen to make helium. This releases a burst of energy, but it also releases two high-energy neutrons. For energy research, this causes problems: the high volume of fast-travelling neutrons, known as a neutron flux, bombards the walls of the reactor, requiring the careful choice of materials; the particles need to be slowed down to remove their energy; and thick concrete shielding is needed to protect humans from the radiation they produce.
Neutrons have their uses, however, and a few yards away from the massive walls of the JET building, a more modestly sized edifice houses a fledgling company, Tokamak Solutions, which is aiming to develop fusion as a commercial prospect to exploit these enigmatic elementary particles. Using fusion as a neutron source, the company argues, could push the technique into the industrial mainstream well ahead of its use in energy, making it a concrete fact rather than a research aspiration and giving it more credibility to governments and financiers.
Fusion-derived neutrons could have applications in medicine and in the treatment of radioactive nuclear waste, said chief executive David Kingham. And bringing fusion into industry could also, he claimed, help to solve some of the pressing technical problems that are currently besetting fusion energy. The company recently secured £170,000 in funds from a group of investors to develop its ideas.
“One serious and long-term side effect of Fukushima, I think, will be a world shortage of medical isotopes”DAVID KINGHAM, TOKOMAK SOLUTIONS
’There’s been a feeling in the past that the challenges of fusion are so great that they can only be justified if you have this enormous potential of fusion for energy,’ Kingham said. Some feel fusion energy is so vital that to explore other potential uses would be a distraction, he added. However, one JET researcher, Russian physicist Mikhail Gryaznevich, was convinced there had to be other applications for fusion and, moreover, developing these could provide valuable data to feed back into fusion energy research.
’Mikhail started thinking about neutrons, and particularly neutrons to deal with nuclear waste,’ said Kingham. Among the wastes that fission reactors produce are heavy metals called actinides: the group of elements that includes uranium and plutonium, but also metals such as americium and neptunium. The radioactivity of these elements is very long lasting, with half-lives in excess of 10,000 years. However, it’s known that bombarding them with energetic neutrons forces the radioactive nuclei to split, forming other elements whose radioactivity lasts only for years or decades, making them easier to store until the radiation hazard has declined to safe levels.
’Mikhail’s vision is to have a reactor that is useful as a powerful neutron source within five or 10 years, giving us something that can deal with nuclear waste,’ said Kingham. ’But what we’ve worked out over the last year or so is that we could take a series of smaller steps to get there, introducing additional complexity at each stage.’
Tokamak Solutions is aiming to produce ’super-compact’ tokamaks - doughnut-shaped fusion reactors that use magnetic fields to confine and manipulate hot plasma, the gaseous soup of charged particles resulting from stripping the electrons away from atoms.
’We’re looking at spherical tokamaks, which were developed here at Culham,’ said physicist Alan Sykes, co-founder of the company with Kingham and Gryaznevich and a major figure in UK fusion research. ’These are simpler than devices such as JET and ITER, which are big doughnut-shaped reaction vessels. Instead, they use a cylindrical one, and the magnetic coils pass through a vertical tube down its centre. You can imagine the shape of the plasma as a doughnut that’s been squeezed so it’s a sphere, with a small hole in the middle.’
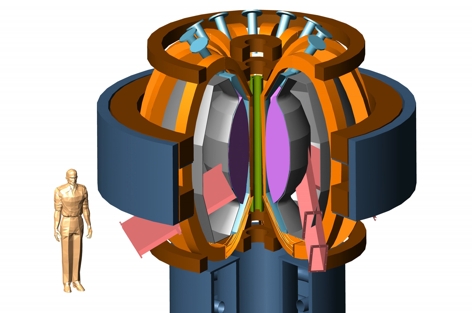
The first spherical tokamak was START (which stands for ’small tight aspect ratio tokamak’), built in 1997 after Sykes’ theoretical work suggested that the spherical configuration would make more efficient use of the magnetic fields. ’We measure the efficiency of a tokamak according to how much plasma pressure you can contain for a unit of magnetic pressure,’ added Sykes. ’Magnetic pressure is expensive - the less of it you need, the better. And when we built START, it turned out we were right. We managed an efficiency three times higher than the previous record and obtained a beautifully defined plasma.’
Tokamak Solutions’ plan is to develop a series of spherical tokamaks, based on the design of START and its successor, MAST (which stands for ’mega-ampere spherical tokamak’). ’Initially, we’d offer a small reactor just designed to produce a plasma, with no fusion,’ said Kingham. ’That would be useful as a research machine for plasma science, and there are about 250 plasma research centres around the world. We think we’d be able to offer these, with a diameter of about 1m, for less than a million dollars.’ This option could include magnets made from high-temperature superconductors, operating at liquid-nitrogen temperature; one of Tokamak Solutions’ investors is Oxford Instruments, a superconductor specialist. This would make it cheaper to run the tokamak, as it would reduce the amount of electricity needed to produce the magnetic fields.
The next stage would be to scale up the reactor to about 3m across - the size of MAST, and about a fifth of the proposed diameter of ITER - and add some equipment to heat the plasma, a key stage in inducing fusion. Although the magnetic fields confine the plasma, fusion cannot occur unless the plasma has energy to overcome the electromagnetic repulsion between the nuclei. Plasma heating methods have been developed at JET and elsewhere, but further development is needed to reduce the cost of the equipment, according to Sykes.
Using a plasma of deuterium, the form of hydrogen containing one proton and one neutron, would produce fusion at a relatively small scale, generating a neutron flux of about 2MW, or 1018 neutrons per second. ’That’s enough power to think about using neutrons in medicine,’ said Kingham. There are several applications here, one of which is the production of radioactive isotopes for nuclear medicine, used for imaging and cancer therapy. Currently, these are produced in five research fission reactors, in the Netherlands, Poland, Belgium, France and South Africa. ’One serious and long-term side effect of Fukushima, I think, will be a world shortage of medical isotopes,’ he added. ’There was already a shortage and that was only alleviated by extensions of lifetime on these reactors.’ Kingham believes that fusion could be a key technology in lifting such shortages.
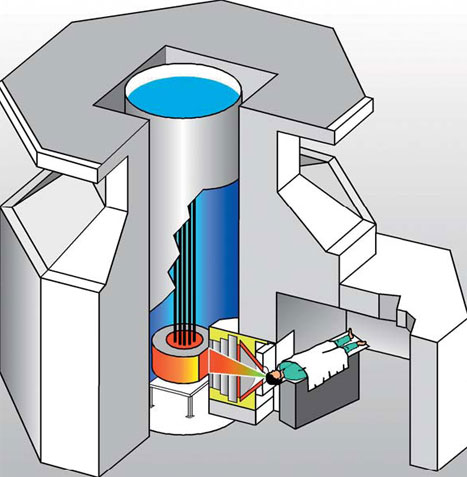
Another possibility is a technique under trial at the Massachusetts Institute of Technology (MIT) and in Otaniemi, Finland, called neutron capture therapy. This involves attaching atoms of boron or gadolinium to molecules that target and bind to tumour cells, then firing a beam of neutrons onto the tumour. The neutrons induce the boron or gadolinium to produce localised radiation, which destroys the cells to which they are attached without affecting neighbouring tissues. Currently, the neutrons are produced by nuclear reactors near MIT and Otaniemi. ’It’s limited by the availability of neutron sources,’ said Kingham. ’But you could use compact tokamaks near enough to clinical facilities, with the right screening and radiological safety, in a small facility.’
The super-compact fusion nuclear sources using tritium could be key to the safer disposal of nuclear waste
The next stage up from this is to use tritium in the tokamak. Tritium-deuterium fusion is much more efficient than deuterium-deuterium and would produce an 80-fold increase in neutron flux; however, tritium is radioactive and requires special handling. ’We’d be running into the same research problems that are affecting projects such as ITER,’ said Kingham. ’Things such as the materials that can withstand the neutron flux for long periods, and systems within the reactor that can use the neutron bombardment to generate tritium from lithium. But spherical tokamaks are a very good research tool to investigate these problems. We think that, with the experience of the earlier stages, we’d be in a good position to work on that. In a way, a logical customer for a powerful neutron source would be ITER and its successor organisations, to test their materials, develop their systems and gain experience in running fusion machines.’
The super-compact fusion neutron sources using tritium could be key to the safer disposal of nuclear waste and a vital part of another proposed nuclear technology: the fusion-fission hybrid reactor. In current fission reactors, a critical mass of fissile material needs to be within the reactor at all times, as this provides a neutrons flux that triggers a fission chain reaction. But if a fusion reactor is used as a neutron source, it could generate the neutron flux inside a fission reactor containing a smaller amount of fissile material, far below the critical mass. These subcritical reactors could reduce the risk of running a fission reactor, and the neutron bombardment would destroy waste elements as they are produced, as well as providing a way to treat existing nuclear waste.
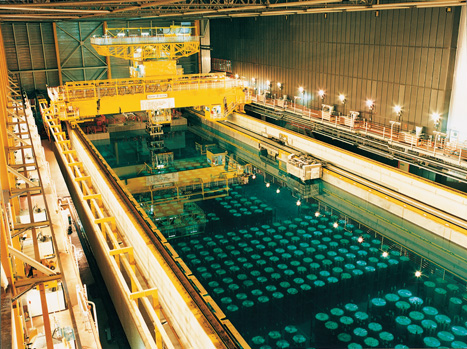
’We are not creating new technology to capture neutrons,’ said Gryaznevich. ’This is established technology that needs to be adapted. These reactors could help us to solve the problems of fusion energy. We just need to build the tokamaks. We know how to do that, and their use could provide us with the funding and the experience we need.’
what’s next
waste matter
Manchester University’s Tim Abram on whether to store or treat long-lived waste to protect future generations
Transmutation reactors have been a target for nuclear technologists since the 1950s, both as breeder reactors, generating their own nuclear fuel, and for destroying waste. However, Tim Abram, director of the Centre for Nuclear Energy Technology at Manchester University, said their development has been difficult.
’Some of the waste generated in reactors is highly radioactive, but that activity dies away quite quickly. Other waste, such as actinides, is less radioactive, but it lasts a very long time. The reason to develop transmutation reactors is that some people think it’s immoral to leave long-lived waste to affect future generations.’
Although actinides are classed as high-level waste, they don’t represent a major hazard, said Abram. ’None are absorbed by the body; the activity is quite low; and they aren’t very mobile. Millions of years ago, uranium contained a larger proportion of the fissile isotope than it does today, and in some places it formed natural nuclear reactors. Those generated actinides, and they haven’t moved from where they were formed. That indicates that they could be stored safely, and that calls into question the idea of this very expensive technology to treat them.’
Subcritical reactors have also been suggested, using particle accelerators to generate the neutron flux. ’There are two problems,’ said Abrams. ’The first is that regulators have to take the pessimistic view and assume the neutron beam can’t be turned off, which means that the fission reactor needs all the same safety systems as a critical-mass reactor. That increases the complexity and the cost. The second is that both of these systems use complex and difficult technology.’
Abrams thinks that the same provisos would apply to fusion-fission reactors. ’But this is really a political choice,’ he said. ’At the moment, the political will is behind storage. But we know that if we’re going to continue using nuclear, as some point we will have to use breeder reactors, because there isn’t enough fissile uranium to keep running conventional reactors. That could open up the path for these new technologies.’
indepth
building up energy
Two important projects, namely the ITER tokomak and the US National Ignition Facility, are currently in the pipeline
Two important projects, namely the ITER tokomak and the US National Ignition Facility, are currently in the pipelineThe fusion community’s attention is currently focused on two projects: the magnetic confinement (i.e. tokamak) ITER and the US National Ignition Facility (NIF) at the Lawrence Livermore National Laboratory in California. The NIF is investigating a different form of fusion, inertial confinement, in which small amounts of fusion fuel are compressed by using powerful lasers to vaporise a capsule surrounding the fuel.
The technology is somewhat further ahead than magnetic confinement because it is based more closely on the first demonstration of man-made fusion, in hydrogen bombs.
ITER is closely based on JET, whose tritium handling facility makes it the only tokamak in the world capable of performing fusion. In order to make a self-sustaining fusion reaction producing more energy than it takes to keep the reaction going, it needs to be twice as large as JET, 19.4m in diameter, and will use low-temperature superconductors to form its magnets. These are currently the source of technical problems, as the superconducing material is proving more brittle than expected in bulk.
The NIF is working towards the first demonstration of net energy production from fusion. It began firing laser pulses in 2009 and has now begun working on hydrogen-filled targets, generating small neutron fluxes. The team plans to start working on deuterium-tritium fuel mixtures this year and hopes to demonstrate net energy gain in 2012.
Five ways to prepare for your first day
If I may add my own personal Tip No. 6 it goes something like this: From time to time a more senior member of staff will start explaining something...