Brain power: UK engineers lead the way in childhood epilepsy treatment
A UK developed medical implant is at the heart of a pioneering clinical trial that could transform the treatment of severe childhood epilepsy. Jon Excell reports.
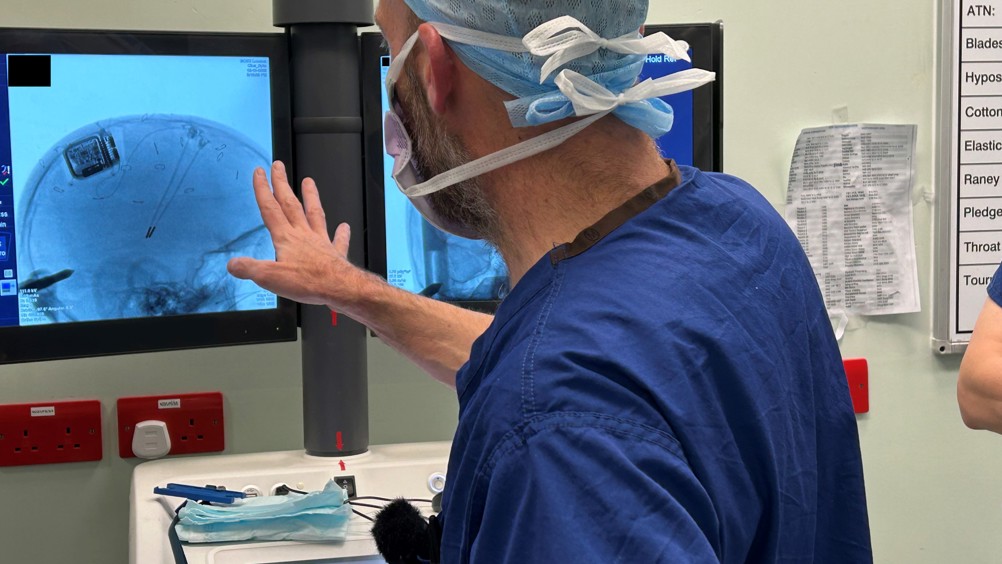
Earlier this year, UK clinicians hailed a potentially huge breakthrough in the treatment of childhood epilepsy, with the news that a clinical trial of a pioneering brain implant has apparently transformed the life of a British teenager suffering from one of the severest forms of the illness.
According to initial reports, since having the UK-developed Picostim device fitted last October at London’s Great Ormond Street Hospital, thirteen-year-old Oran Knowlson (who suffers from a hitherto treatment resistant form of epilepsy known as Lennox-Gastaut syndrome) has seen an 80 per cent reduction in daytime seizures, which previously sometimes occurred hundreds of times a day.
The development is the latest in a series of trials taking place through the CADET project (The Children’s Adaptive Deep brain stimulation for Epilepsy Trial), a three-year long initiative led by UCL Great Ormond Street Institute of Child Health and Great Ormond Street Hospital aimed at exploring the effectiveness of deep brain stimulation for children with epilepsy.
Register now to continue reading
Thanks for visiting The Engineer. You’ve now reached your monthly limit of premium content. Register for free to unlock unlimited access to all of our premium content, as well as the latest technology news, industry opinion and special reports.
Benefits of registering
-
In-depth insights and coverage of key emerging trends
-
Unrestricted access to special reports throughout the year
-
Daily technology news delivered straight to your inbox
Water Sector Talent Exodus Could Cripple The Sector
Maybe if things are essential for the running of a country and we want to pay a fair price we should be running these utilities on a not for profit...